这是一份nottingham诺丁汉大学PHYS4014作业代写的成功案例

Here we see that a complex $\chi(\omega)$ results from a real $\chi\left(t-t^{\prime}\right)$. Inserting
$$
\frac{1}{\varepsilon_{0}} \boldsymbol{P}(\omega)=\int_{-\infty}^{+\infty} \mathrm{e}^{\mathrm{i} \omega t}\left[\int_{-\infty}^{t} \chi\left(t-t^{\prime}\right) \boldsymbol{E}\left(t^{\prime}\right) \mathrm{d} t^{\prime}\right] \mathrm{d} t .
$$
Introducing
$$
1=\mathrm{e}^{-\mathrm{i} \omega t^{\prime}} \mathrm{e}^{\mathrm{i} \omega t^{\prime}}
$$
in the inner integral and rearranging the terms gives
$$
\frac{1}{\varepsilon_{0}} \boldsymbol{P}(\omega)=\int \boldsymbol{E}\left(t^{\prime}\right) \mathrm{e}^{\mathrm{i} \omega t^{\prime}}\left[\int \chi\left(t-t^{\prime}\right) \mathrm{e}^{\mathrm{i} \omega\left(t-t^{\prime}\right)} \mathrm{d} t\right] \mathrm{d} t^{\prime}=\chi(\omega) \boldsymbol{E}(\omega) .
$$
This is identical
With the knowledge of (6.11) we can apply Cauchy’s theorem, which connects the real and imaginary parts of the Fourier transforms of analytic functions. This theorem leads us to
$$
\varepsilon_{1}(\omega)-1=\frac{1}{\pi} P \int_{-\infty}^{+\infty} \frac{\varepsilon_{2}\left(\omega^{\prime}\right)}{\omega^{\prime}-\omega} \mathrm{d} \omega^{\prime}
$$
and
$$
\varepsilon_{2}(\omega)=-\frac{1}{\pi} \int_{-\infty}^{+\infty} \frac{\varepsilon_{1}\left(\omega^{\prime}\right)-1}{\omega^{\prime}-\omega} d \omega^{\prime}
$$
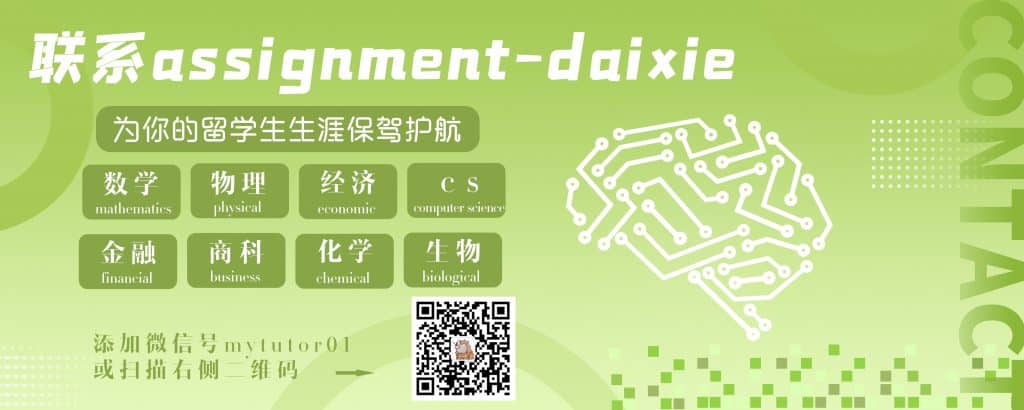
PHYS4014 COURSE NOTES :
and cyclic permutations of the indices $V_{u c}$ is the volume of the unit cell given by
$$
V_{\mathrm{uc}}=\boldsymbol{a}{1}\left(\boldsymbol{a}{2} \times \boldsymbol{a}{3}\right) . $$ A general translation vector of the reciprocal lattice is usually called $\boldsymbol{G}$ $$ \boldsymbol{G}=l{1} \boldsymbol{b}{1}+l{2} \boldsymbol{b}{2}+l{3} \boldsymbol{b}{3} \quad l{i}=0, \pm 1, \pm 2, \ldots \quad i=1,2,3 .
$$
Without trying to be complete, we give some properties of the reciprocal lattice and its connections with the real one.
Every periodic function in real space which is sufficiently smooth and has a periodicity given by $f(\boldsymbol{r}+\boldsymbol{R})=f(\boldsymbol{r})$ and $\boldsymbol{R}$ defined by (7.6) can be written as a Fourier series summing over all vectors of the reciprocal lattice
$$
f(r)=\sum_{G} f_{G} \mathrm{e}^{\mathrm{i} G r}
$$
with
$$
f_{G}=V_{u c}^{-1} \int_{u c} f(\boldsymbol{r}) \mathrm{e}^{-\mathrm{i} \boldsymbol{G r}} \mathrm{d} \tau .
$$
The scalar product of $R$ and $G$ always fulfills
$$
\boldsymbol{R} \cdot \boldsymbol{G}=2 \pi m ; \quad m=0, \pm 1, \pm 2, \ldots
$$