Assignment-daixieTM为您提供格拉斯哥大学University of Glasgow ALGEBRA MATHS4072代数代写代考和辅导服务!
Instructions:
Algebraic structures, such as groups, rings, and fields, are fundamental concepts in abstract algebra that have important applications in many areas of mathematics and beyond.
Group theory is the study of the algebraic structure called a group, which is a set of elements with a binary operation that satisfies certain axioms. Group theory has applications in many areas of mathematics, including number theory, geometry, and topology.
Ring theory is the study of rings, which are algebraic structures that generalize the properties of integers. Rings are important in algebraic geometry, number theory, and coding theory.
Field theory is the study of fields, which are algebraic structures that extend the properties of rational numbers. Fields are important in algebraic geometry, number theory, and physics.
The construction and application of quotient or factor groups and rings is an important aspect of algebraic structures. Homomorphisms between rings and groups are also essential in the study of algebraic structures.
Overall, this course will provide a solid foundation in the basic concepts and techniques of abstract algebra, which will be useful in many areas of mathematics and beyond.
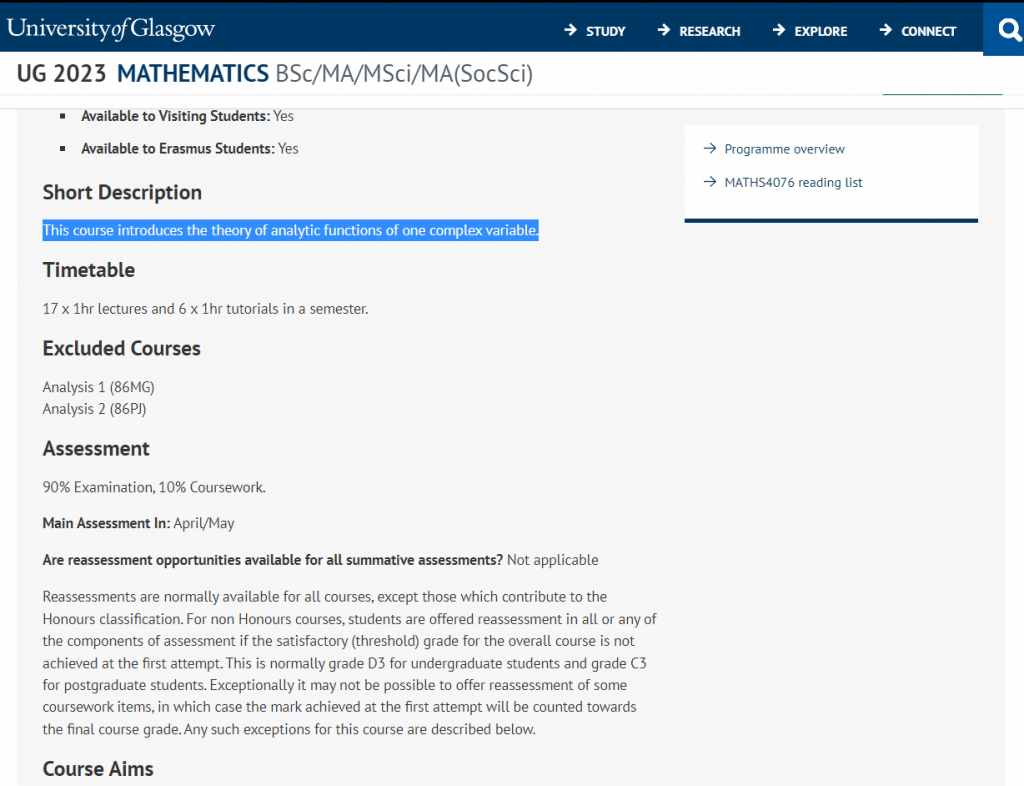
Let $[n]$ denote the totally ordered set $\{0,1, \ldots, n\}$. Let $\phi:[m] \rightarrow[n]$ be an order preserving function (so that if $i \leq j$ then $\phi(i) \leq \phi(j)$ ). Identifying the elements of $[n]$ with the vertices of the standard simplex $\Delta^n, \phi$ extends to an affine $\operatorname{map} \Delta^m \rightarrow \Delta^n$ that we also denote by $\phi$. Give a formula for this map in terms of barycentric coordinates: If $\phi\left(s_0, \ldots, s_m\right)=\left(t_0, \ldots, t_n\right)$, what is $t_j$ as a function of $\left(s_0, \ldots, s_m\right) ?$
Let $\Delta^n$ be the standard $n$-simplex, that is, $\Delta^n = {(x_0, x_1, \ldots, x_n) \in \mathbb{R}^{n+1} : x_i \geq 0, \sum_{i=0}^n x_i = 1}$. We will use the barycentric coordinates for the points in $\Delta^n$, which are defined as follows: for $0 \leq i \leq n$, let $e_i \in \Delta^n$ be the point with $x_i = 1$ and $x_j = 0$ for $j \neq i$. Then any point $p \in \Delta^n$ can be written uniquely as a convex combination of the $e_i$’s, that is, $p = \sum_{i=0}^n \lambda_i e_i$, where $\lambda_i \geq 0$ and $\sum_{i=0}^n \lambda_i = 1$.
Now let $\phi: [m] \to [n]$ be an order-preserving function, and let $\phi:\Delta^m \to \Delta^n$ be the affine map that extends $\phi$ to the standard simplices. We want to express $\phi(p)$ in terms of the barycentric coordinates of $p = \sum_{i=0}^m \lambda_i e_i$.
Note that $\phi(e_i)$ is a point in $\Delta^n$, so we can write $\phi(e_i)$ as a convex combination of the $e_j$’s. Let $\phi(e_i) = \sum_{j=0}^n a_{ij} e_j$, where $a_{ij} \geq 0$ and $\sum_{j=0}^n a_{ij} = 1$. Then for any $p \in \Delta^m$, we have \begin{align*} \phi(p) &= \phi\left(\sum_{i=0}^m \lambda_i e_i\right) \ &= \sum_{i=0}^m \lambda_i \phi(e_i) \ &= \sum_{i=0}^m \lambda_i \sum_{j=0}^n a_{ij} e_j \ &= \sum_{j=0}^n \left(\sum_{i=0}^m \lambda_i a_{ij}\right) e_j. \end{align*}
Let $t_j = \sum_{i=0}^m \lambda_i a_{ij}$, so we have $\phi(p) = \sum_{j=0}^n t_j e_j$. Since $e_j$ is the point with $x_j = 1$ and $x_i = 0$ for $i \neq j$, we have $t_j = \phi(\lambda_j)$, where $\lambda_j$ is the $j$th barycentric coordinate of $p$. Therefore, we have
$t_j=\sum_{i=0}^m \lambda_i a_{i j}$,
where $a_{ij}$ is the $j$th barycentric coordinate of $\phi(e_i)$.
(a) Write $\pi_0(X)$ for the set of path-components of a space $X$. Construct an isomorphism $$ \mathbb{Z} \pi_0(X) \rightarrow H_0(X) $$
Let $X$ be a topological space. We denote by $\pi_0(X)$ the set of path-components of $X$. That is, for any two points $x,y\in X$, we say that $x$ and $y$ are in the same path-component if there exists a path $\gamma:[0,1]\to X$ such that $\gamma(0)=x$ and $\gamma(1)=y$. The set $\pi_0(X)$ is a partition of $X$ into disjoint subsets, and each subset is a path-connected subspace of $X$.
Now, we construct an isomorphism between $\mathbb{Z} \pi_0(X)$ and $H_0(X)$. Let $\mathbb{Z}\pi_0(X)$ be the free abelian group generated by the set $\pi_0(X)$, and let $\phi:\mathbb{Z}\pi_0(X)\to H_0(X)$ be the homomorphism defined as follows. For each path-component $[x]\in \pi_0(X)$, choose a point $x\in [x]$, and define $\phi([x])=[x]$ to be the class in $H_0(X)$ represented by the point $x$. We extend $\phi$ to all of $\mathbb{Z}\pi_0(X)$ by linearity.
We claim that $\phi$ is an isomorphism. To see this, we construct its inverse. Let $\psi:H_0(X)\to \mathbb{Z}\pi_0(X)$ be the homomorphism defined by sending each class $[x]\in H_0(X)$ to the generator $[x]\in \mathbb{Z}\pi_0(X)$ corresponding to the path-component containing $x$. That is, if $[x]\in H_0(X)$, then there exists a chain $\sum_i n_i \sigma_i$ with $\sigma_i:\Delta^0\to X$ such that $\partial (\sum_i n_i \sigma_i)=[x]$, and we define $\psi([x])=[\sigma_i(0)]\in \mathbb{Z}\pi_0(X)$. Again, we extend $\psi$ to all of $H_0(X)$ by linearity.
It is easy to see that $\psi$ is well-defined and that $\phi$ and $\psi$ are inverses of each other, so $\phi$ is an isomorphism.
Let $A$ be a chain complex (of abelian groups). It is acyclic if $H(A)=0$, and contractible if it is chain-homotopy-equivalent to the trivial chain complex. Prove that a chain complex is contractible if and only if it is acyclic and for every $n$ the inclusion $Z_n A \hookrightarrow A_n$ is a split monomorphism of abelian groups.
First, let’s assume that the chain complex $A$ is contractible. This means that there exists a chain map $s:A\rightarrow A$ (called a homotopy) such that $ds+sd=\mathrm{id}_A$, where $d$ is the differential of $A$. We can then define a chain map $r:A\rightarrow A$ by $r_n = \frac{1}{2}(1+s)$, which is well-defined because $s$ is a chain map. It’s easy to check that $r$ is also a chain map, and that $sr=\mathrm{id}_A$. This shows that $A$ is chain-homotopy-equivalent to the trivial chain complex.
Next, let’s assume that $A$ is acyclic and that the inclusion $Z_n A\hookrightarrow A_n$ is a split monomorphism for every $n$. Since $A$ is acyclic, we have $H_n(A)=0$ for all $n$. It remains to show that $A$ is chain-homotopy-equivalent to the trivial chain complex.
For each $n$, let $s_n:A_n\rightarrow A_{n+1}$ be a map such that $ds_n+s_{n-1}d=\mathrm{id}{A_n}$ (recall that we set $s{-1}=0$). Such a map exists because the inclusion $Z_n A\hookrightarrow A_n$ is a split monomorphism. In fact, we can take $s_n$ to be the composite of the projection $A_{n+1}\rightarrow Z_{n+1}A$ (which exists because of the split monomorphism) and the inclusion $Z_{n+1}A\hookrightarrow A_{n+1}$.
We claim that $s={s_n}$ is a chain homotopy between $A$ and the trivial chain complex. To see this, we need to show that $ds+sd=\mathrm{id}A$. We have: \begin{align*} (ds+sd)n &= d_ns{n-1}+s{n-1}d_n \ &= (s_{n-1}d-d_{n-1}s_n)+s_{n-1}d_n \ &= s_{n-1}(d_n+d_{n-1}) \ &= s_{n-1}^2 \ &= \frac{1}{4}(1+s_{n-1})^2 \ &= \frac{1}{4}(1+2s_{n-1}+s_{n-1}^2) \ &= \frac{1}{4}(1+2s_{n-1}+(1+s_{n-2})s_n) \ &= \frac{1}{4}(2s_n+s_{n-1}s_n+s_{n-2}s_n) \ &= \frac{1}{4}(2s_n+s_{n-1}d_n+s_{n-2}d_{n-1}) \ &= \frac{1}{4}(2s_n+d_{n-1}s_n+d_ns_{n-1}) \ &= \frac{1}{4}(2s_n+d_ns_{n-1}+s_{n-1}d_n) \ &= \frac{1}{4}(2s_n+\mathrm{id}{A_n}) \ &= r_n, \end{align*}
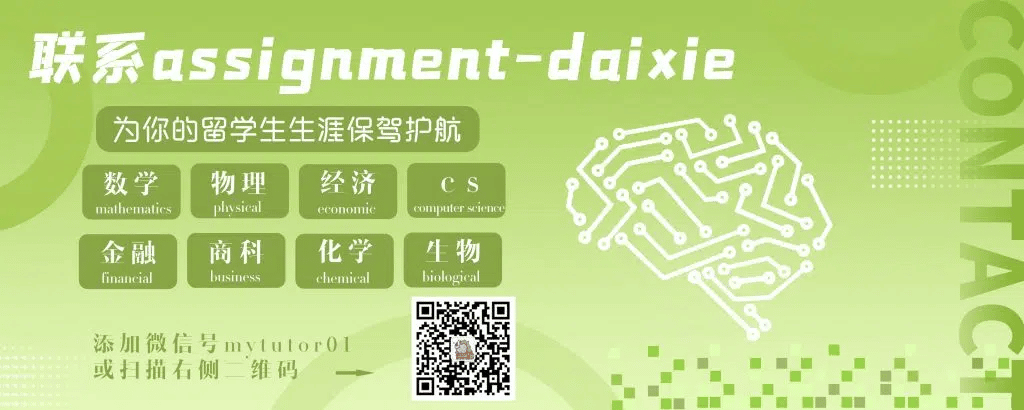