Assignment-daixieTM为您提供利物浦大学University of Liverpool NUMERICAL METHODS FOR APPLIED MATHEMATICS MATH226应用数学的数值方法代写代考和辅导服务!
Instructions:
Applied mathematics often deals with complex problems that cannot be solved exactly using analytical methods. In such cases, numerical methods provide an alternative approach to solve these problems using computers. These methods involve approximating solutions by breaking down the problem into smaller parts and performing iterative calculations until a desired level of accuracy is achieved.
Numerical methods are commonly used in a variety of fields, such as physics, engineering, finance, and biology, to solve problems that involve real-world data. For example, in finance, numerical methods are used to calculate the value of complex financial derivatives, while in biology, these methods are used to simulate the behavior of large-scale biochemical networks.
One of the key advantages of numerical methods is their ability to handle complex problems that would be impractical or impossible to solve by hand. These methods allow for the efficient solution of problems that involve large amounts of data, complex equations, or nonlinear relationships.
However, numerical methods are not without their drawbacks. One major issue is the propagation of errors, where small errors in the initial data or calculations can lead to significant errors in the final results. To mitigate this issue, it is essential to carefully analyze the sources of error and design algorithms that minimize their impact.
In this module, you will learn about various numerical methods, including methods for finding roots, approximating integrals, and interpolating data. You will examine the advantages and disadvantages of different approaches in terms of accuracy and efficiency, and you will learn how to write computer programs to handle calculations automatically. By the end of the module, you will have a solid understanding of numerical methods and be able to apply them to solve complex problems in various fields.
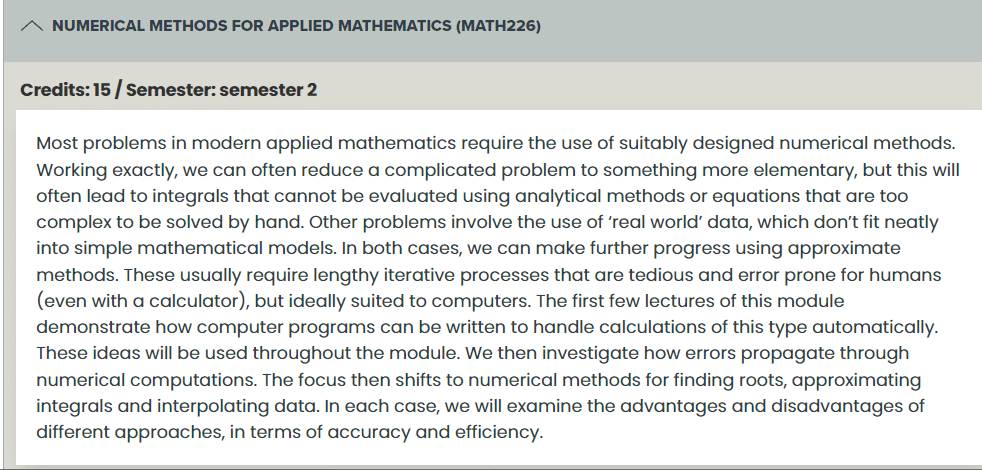
Create a real $4 \times 4$ matrix with rank 2 . Propose a vector $\mathbf{b} \in \mathbb{R}^4$ such that the system of equations $\mathbf{A x}=\mathbf{b}$ has a family solutions. What is that family of solutions? Propose a vector $\mathbf{b} \in \mathbb{R}^4$ such that the system of equations $\mathbf{A x}=\mathbf{b}$ has no solutions. Explain why this is the case.
There are many possible answers. A diagonal matrix with rank 2 would be:
$$
\left(\begin{array}{llll}
1 & 0 & 0 & 0 \
0 & 1 & 0 & 0 \
0 & 0 & 0 & 0 \
0 & 0 & 0 & 0
\end{array}\right)
$$
A value of $\mathbf{b}=(1,0,0,0)$ would give a family of solutions $\mathbf{x}=(1,0, a, b)$ where $a$ and $b$ are arbitrary. A value of $\mathbf{b}=(0,0,1,0)$ would give no solution since this vector is not in the range space of the matrix.
Write a MATLAB ${ }^6$ function that takes advantage of this sparsity pattern to compute the product of $\mathbf{S}$ with a vector. Your function should take as an input the fluxes associated with each reaction in the cycle and return the rate of change for the concentration of each species in the cycle. Be sure that your function does not compute the stoichiometry matrix explicitly.
function $y=$ sparse_mult $(x)$
$$
y(1)=-x(1)+x(\text { end })
$$
for $i=2:$ length $(x)$
$$
y(i)=x(i-1)-x(i) ;
$$
end;
Develop an expression for the characteristic polynomial of the $\mathrm{N}$ component stoichiometry matrix, S. The roots represent the eigenvalues of $\mathbf{S}$.
$$
\operatorname{det}(\mathbf{S}-\lambda \mathbf{I})=(-1-\lambda) M_{11}+(-1)^{N-1} M_{1 N}=0
$$
Because the minors are determinants of diagonal/upper triangular matrices, these are simple to calculate: $M_{11}=(-1-\lambda)^{N-1}, M_{1 N}=1$. Therefore the secular polynomial satisfies $(-1-\lambda)^N+(-1)^{N-1}=0$.
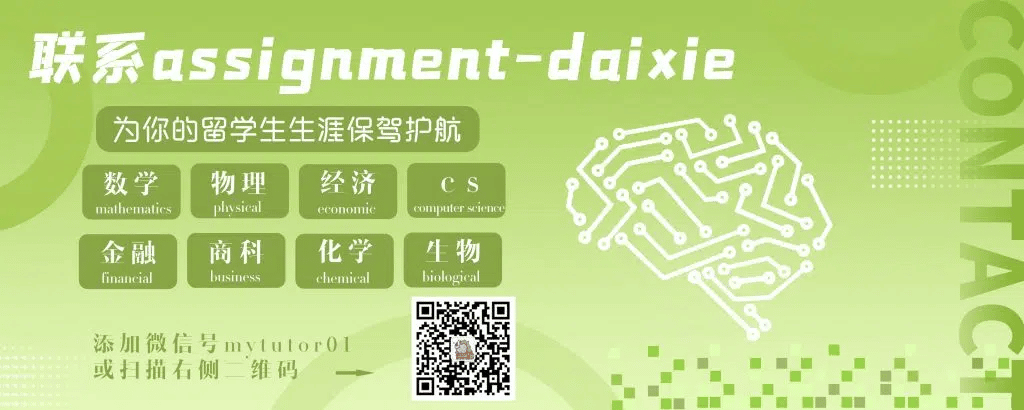