Assignment-daixieTM为您提供利物浦大学University of Liverpool NEWTONIAN MECHANICS MATH122牛顿力学代写代考和辅导服务!
Instructions:
Newtonian mechanics, also known as classical mechanics, is a branch of physics that deals with the study of motion and its causes. It was founded by Sir Isaac Newton in the 17th century and is based on three laws known as Newton’s laws of motion.
Newton’s first law of motion states that an object at rest will remain at rest, and an object in motion will continue in motion with a constant velocity unless acted upon by an external force. This law is also known as the law of inertia.
Newton’s second law of motion states that the force acting on an object is equal to the mass of the object times its acceleration. Mathematically, F=ma, where F is the force, m is the mass, and a is the acceleration.
Newton’s third law of motion states that for every action, there is an equal and opposite reaction. This law implies that forces always occur in pairs.
In addition to these laws, Newtonian mechanics also includes the concepts of energy, work, and momentum. The laws of conservation of energy, work-energy theorem, and conservation of momentum are also important principles in this branch of physics.
Newtonian mechanics has been instrumental in the development of modern physics, and its principles are still widely used in many fields of science and engineering.
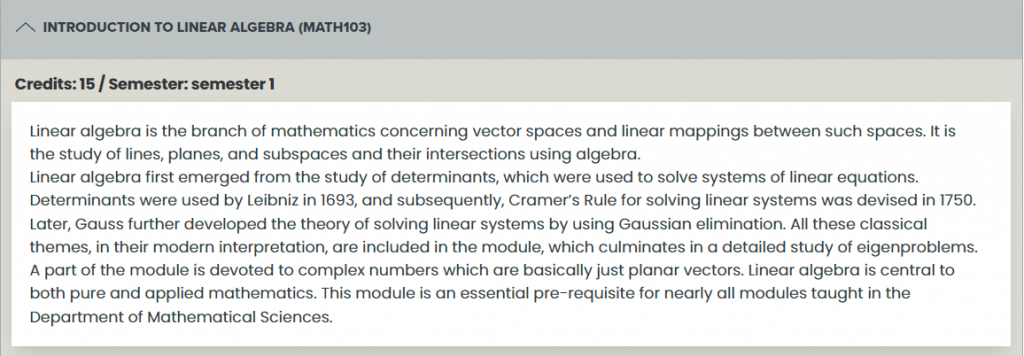
A rocket in zero gravitational field has a mass of $m_{r, i}=2.81 \times 10^7 \mathrm{~kg}$, which is the sum of the mass of the fuel $m_{f, i}=2.46 \times 10^7 \mathrm{~kg}$ and the dry mass of the rocket (empty of fuel) $m_{r, d} \equiv m_{r, i}-m_{f, i}=0.35 \times 10^7 \mathrm{~kg}$. The fuel is ejected at a speed $u=3000$ $\mathrm{m} / \mathrm{s}$ relative to the rocket. The total burn time is $510 \mathrm{~s}$ and the fuel is burned at a constant rate. (a) What is the final speed $v_f$ of the rocket in meters/second after all the fuel is burned assuming it starts from rest?
The total mass of the rocket decreases during the burn due to the ejection of fuel. The change in velocity of the rocket is given by the rocket equation:
$\Delta v=v_{f}-v_{i}=u \ln \left(\frac{m_{r, i}}{m_{r, f}}\right)$
where $v_i = 0$ is the initial velocity of the rocket, $v_f$ is the final velocity of the rocket, $m_{r,i}$ is the initial mass of the rocket, $m_{r,f}$ is the final mass of the rocket, and $u$ is the relative velocity of the ejected fuel. At the end of the burn, all the fuel has been ejected, so the final mass of the rocket is the dry mass $m_{r,d}$:
$m_{r, f}=m_{r, d}=0.35 \times 10^7 \mathrm{~kg}$
Thus,
$\Delta v=u \ln \left(\frac{m_{r, i}}{m_{r, d}}\right)=3000 \mathrm{~m} / \mathrm{s} \ln \left(\frac{2.81 \times 10^7 \mathrm{~kg}}{0.35 \times 10^7 \mathrm{~kg}}\right) \approx 7974.4 \mathrm{~m} / \mathrm{s}$
Therefore, the final speed of the rocket is $v_f = \Delta v = 7974.4$ m/s.
(b) Now suppose that the same rocket burns the fuel in two stages, expelling the fuel in each stage at the same relative speed $u=3000 \mathrm{~m} / \mathrm{s}$. In stage one, the available fuel to burn is $m_{f, 1, i}=2.03 \times 10^7 \mathrm{~kg}$ with burn time $150 \mathrm{~s}$. The total mass of the rocket after all the fuel in stage 1 is burned is $m_{r, 1, d}=m_{r, i}-m_{f, 1, i}=0.78 \times 10^7$ kg. What is the change in speed after stage one is complete?
In stage one, the initial mass of the rocket is $m_{r,1,i}=m_{r,i}=2.81 \times 10^7 \mathrm{~kg}$ and the initial mass of fuel is $m_{f,1,i}=2.03 \times 10^7 \mathrm{~kg}$. The mass of the rocket after all the fuel in stage 1 is burned is $m_{r,1,f}=m_{r,1,i}-m_{f,1,i}=0.78 \times 10^7$ kg. By the principle of conservation of momentum, the change in velocity of the rocket after stage one is complete is given by:
$\Delta v_1=u\ln \left(\frac{m_{r,1,i}}{m_{r,1,f}}\right)$
where $u=3000 \mathrm{~m}/\mathrm{s}$ is the relative speed of the ejected fuel and $m_{r,1,i}$ and $m_{r,1,f}$ are the initial and final masses of the rocket in stage one, respectively. Substituting the values:
$\Delta v_1=3000 \mathrm{~m}/\mathrm{s} \ln \left(\frac{2.81 \times 10^7 \mathrm{~kg}}{0.78 \times 10^7 \mathrm{~kg}}\right) \approx 3066 \mathrm{~m}/\mathrm{s}$
Therefore, the change in speed after stage one is complete is approximately $3066 \mathrm{~m}/\mathrm{s}$.
(c) Next, the empty fuel tank and accessories from stage one are disconnected from the rest of the rocket. These disconnected parts had a mass $m=1.4 \times 10^6 \mathrm{~kg}$, hence the remaining dry mass of the rocket is $m_{r, 2, d}=2.1 \times 10^6 \mathrm{~kg}$. All the remaining fuel with mass $m_{f, 2, i}=4.3 \times 10^6 \mathrm{~kg}$ is burned during stage 2 with burn time of $360 \mathrm{~s}$. What is the change in speed in meters/second after stage two is complete?
To calculate the change in speed after stage two, we can use the rocket equation, which relates the change in velocity of a rocket to the mass ratio of the rocket before and after the burn, and the effective exhaust velocity of the propellant.
The mass ratio of the rocket before and after stage two is:
$$\frac{m_{i}}{m_{f, 2}}=\frac{m_{r, i}+m_{f, i}+m}{m_{r, 2, d}}=\frac{2.81 \times 10^7 \mathrm{~kg}+2.46 \times 10^7 \mathrm{~kg}+1.4 \times 10^6 \mathrm{~kg}}{2.1 \times 10^6 \mathrm{~kg}}=22.95$$
where $m_{i}$ is the initial mass of the rocket (including all the fuel and the dry mass) and $m_{f,2}$ is the final mass of the rocket after stage two (including the dry mass and all the fuel burned in stage two).
The effective exhaust velocity $v_{e}$ is given by:
$$v_{e}=u \ln\frac{m_{i}}{m_{f,2}}=3000 \mathrm{~m/s} \ln\frac{2.81 \times 10^7 \mathrm{~kg}}{2.1 \times 10^6 \mathrm{~kg}+4.3 \times 10^6 \mathrm{~kg}}=2445.5 \mathrm{~m/s}$$
where $u$ is the speed of the ejected fuel relative to the rocket.
Now we can use the rocket equation:
$$\Delta v=v_{e} \ln\frac{m_{i}}{m_{f,2}}=2445.5 \mathrm{~m/s} \ln\frac{2.81 \times 10^7 \mathrm{~kg}}{2.1 \times 10^6 \mathrm{~kg}+4.3 \times 10^6 \mathrm{~kg}}=2434.4 \mathrm{~m/s}$$
Therefore, the change in speed after stage two is $\Delta v = 2434.4$ $\mathrm{m/s}$.
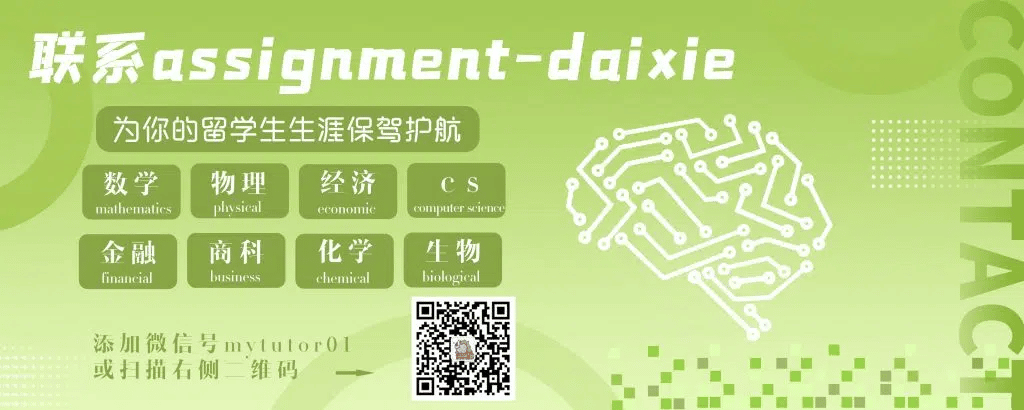